
Rotating ?agella are used to propel bacteria during swimming in water. Each flagellum is a stiff, thin helix attached to a rotary motor that is embedded in a membrane in the cell. As the motor rotates the helix is dragged through the water. This phenomenon superficially resembles the generation of thrust by a boat’s propeller, but actually is quite different. A propeller generates thrust by throwing water backwards; by conservation of momentum, if water is thrown backwards the boat must move forwards. In contrast, a flagellum works by generating different amounts of drag parallel and perpendicular to its centerline. The net result of this is a force imbalance opposite the direction of rotation (for a lefthanded helix): thrust. A careful accounting of the thrust generated by a rotating bundle of several flagella matches with the drag generated by a translocating cell body.
This is an elegantly simple problem in low Reynolds number hydrodynamics, and swimming with a single ?agellum has been rather extensively analyzed, but the corresponding problem in multi-?agellate bacteria is virtually unexplored. This is partly because, for experimental reasons, it is much easier to observe the rotation of a single motor than that of several motors simultaneously. Since there is strong biochemical evidence that all the motors of the cell operate independently, observing one motor at a time should be enough to predict the behavior of an assembly of motors. However, although motors operate independently in theory, flagella attached to motors are seen to operate cooperatively in practice.
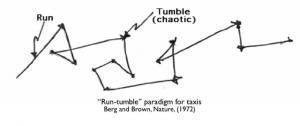
Many bacteria are capable of chemotaxis: sensing chemicals in their environment and swimming preferentially up or down gradients of those chemicals. In most bacteria, chemotaxis is accomplished by actively modulating cells’ “run-tumble” behavior: cells alternate periods of smooth forward swimming (runs) with abrupt reorientations (tumbles). By increasing or decreasing its run length in response to changing levels of chemicals in the environment, a cell can – at least statistically – move up or down a gradient. E. coli motors periodically reverse direction; counterclockwise rotation produces propulsion while clockwise rotation produces reorientation. The cell controls the probability of ccw rotation of a motor by modulating the phosphorylation level of a small signaling protein, CheY. The cell cannot control the rotational direction of each of its motors directly, and probably has no mechanism to sense how many motors it has, much less what each motor is doing at any particular time.
The frequency of tumbling should depend on the number of flagella, since the more flagella a cell has the more likely that one of those flagella is being rotating in reverse, causing a tumble. This implies that, all else being equal, cells with many flagella should have different chemotactic behavior than cells with few flagella. I am building an assay to relate flagellar number to chemotactic ability to test whether such a variability does, in fact, occur in real cells.